Environment & Nature
The science of why hawks are one of nature’s deadliest hunters
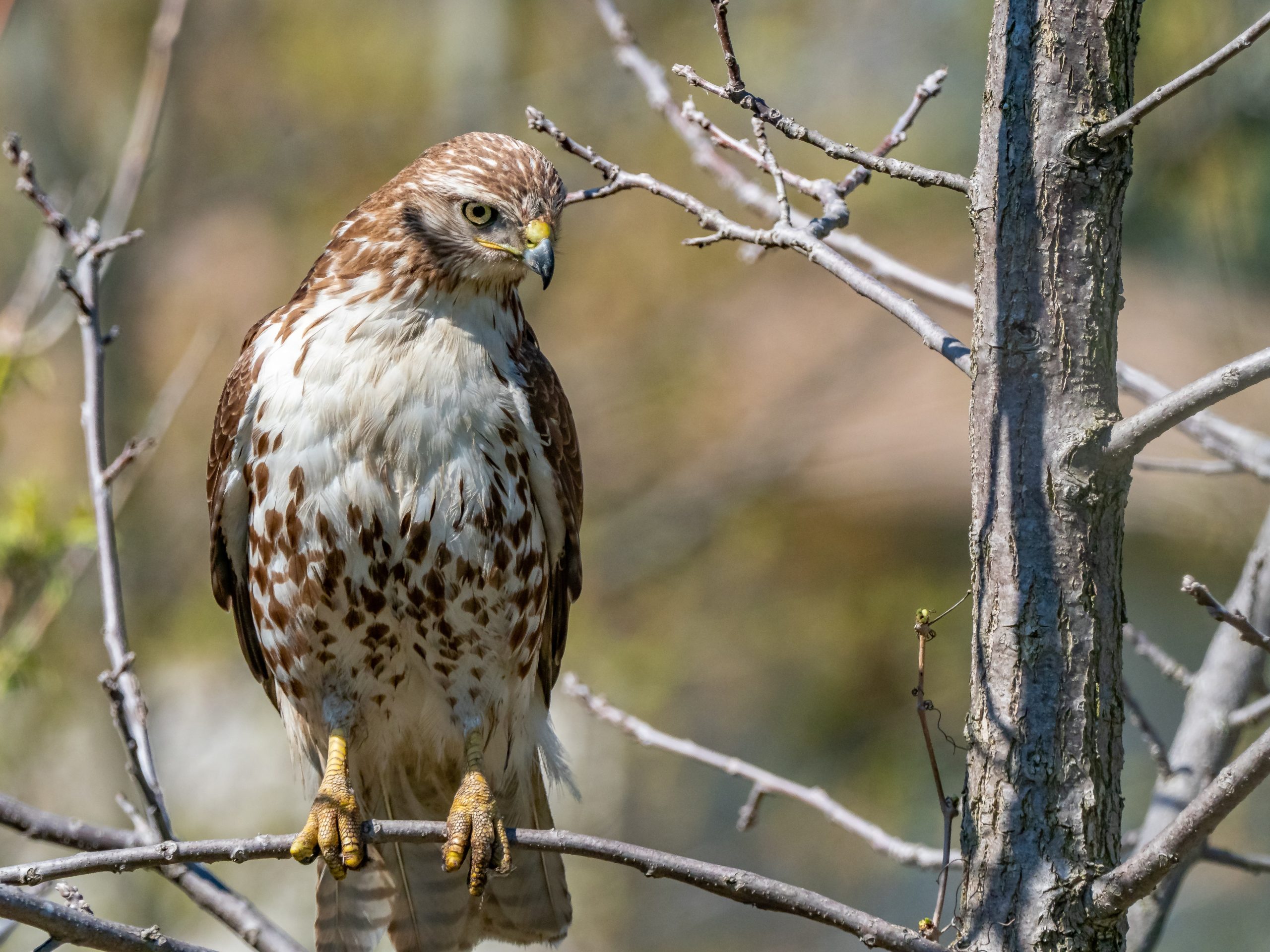
FILE: Red-tailed Hawk (Photo: Patrice Bouchard/Unsplash)
The sight of hundreds of thousands of bats streaming from their roost at dusk is one of nature’s great spectacles. Swarms can be so dense they resemble rising smoke at a distance. But the aerial antics of the birds of prey that hunt them are just as astonishing.
Studying these behaviours in a remote corner of the Chihuahuan Desert (which stretches from the southwest US to Mexico) has been a highlight of my almost 25-year career as a biologist studying animal flight. My team’s study was done in collaboration with bat scientist Laura Kloepper (University of New Hampshire).
Prey animals often find safety in numbers, and bats are no exception. Moving in a group dilutes an individual’s risk of being attacked. It can also confuse the predator and make it harder for them to track a target. This is called the confusion effect. Humans get disorientated in this way by large groups of objects and animals too. Swainson’s hawks tend to attack the Mexican free-tailed bats they feed on as the bats emerge in swarms from their cave. The bats fly much slower as they leave their cave compared to open airspace (they can reach speeds of nearly 100 mph if the area is clear).
My team found the hawks seemed unfazed by the confusion effect.
Seeing clearly
How do hawks steer clear of the confusion effect that bewilders us humans when watching a swarm? To answer this question, we filmed the hawks as they plunged into the stream of bats flowing from a cathedral-like cave. High-definition video cameras placed strategically around the mouth of the bat cave allowed my team to reconstruct the 3D trajectories of the hawks and the bats they attacked. But recreating a behaviour is only the first step towards understanding its mechanics. Next, my team analysed how the hawks steered their line of attack.
We used a computer simulation approach that we had first developed in 2017 to study the attack behaviours of peregrine falcons. This method uses a set of mathematical formulae called differential equations to simulate the birds’ behaviour.
Our previous work had shown that the attack behaviours of falcons are steered in a similar way to guided missiles, using a technique called proportional navigation. To understand how this works, imagine yourself as an aerial predator looking at your prey while closing in on it at speed. Your prey may try to evade you. But if you turn at a rate proportional to the rate at which the compass bearing of your prey changes, then you will take a path that gives you the best chance of intercepting your quarry.
A bird using this technique to chase lone prey will naturally follow the twists and turns of its target. But the hawks we filmed attacking the swarm did not appear to respond to the individual motions of the bats they grabbed. In fact, the hawks simply turned on a near constant radius into the swarm, flying along an almost circular arc. The birds were steering towards a fixed point in the swarm instead of singling out a bat.
This strategy would be hopeless against an erratic solo target, but has a good prospect of success against a dense gathering of prey. It would not be surprising if a bat happened to be hit by an arrow shot at random into the swarm, and the same holds for a diving raptor. The hawks’ success at avoiding the confusion effect may be partially explained by the fact they can circumvent the complication of tracking a target on approach.
Closing in
Even so, catching a bat in your talons is not easy. It’s a complicated process that more often than not ended in failure for the hawks. So how did the hawks select which bat to grab once they had closed in on the swarm?
We used graphing software to trace how the line of sight drawn from the attacker to its target varied through the attack. This analysis revealed a clear answer: the direction of the line of sight to the target remained almost constant on all the attacks. It varied least on those that ended in a successful catch.
This geometry holds true for any pair of objects on a collision course. Mariners are taught to spot a constant bearing to avoid crashes with other boats and drivers use it intuitively to merge safely onto a busy road. The colony of up to 900,000 bats that we studied emerged en masse in the space of just a few minutes. For a predator plunging into this dense a swarm, there are so many targets at least one will be on a collision course. The difficult bit is to identify and catch it.
Whereas a stationary onlooker will perceive the whole swarm to be in motion, the geometry of a collision means that a moving observer will see anything it is on course to hit as stationary. Swarms, flocks, and shoals that look confusing to our own eyes seem more orderly to a mobile predator taking the plunge.
The same will hold true for any attacker, whether a shark ambushing a school of tuna, or a drone defending against a swarm of incoming drones. Our results have implications for understanding how other predators avoid a confusion effect, and even for designing autonomous air defences. Above all, our findings are a neat example of the clever ways in which nature adapts to solve difficult challenges.
Graham Taylor, Professor of Mathematical Biology, University of Oxford
This article is republished from The Conversation under a Creative Commons license. Read the original article.